Could energy efficiency be quantum computers’ greatest strength yet?
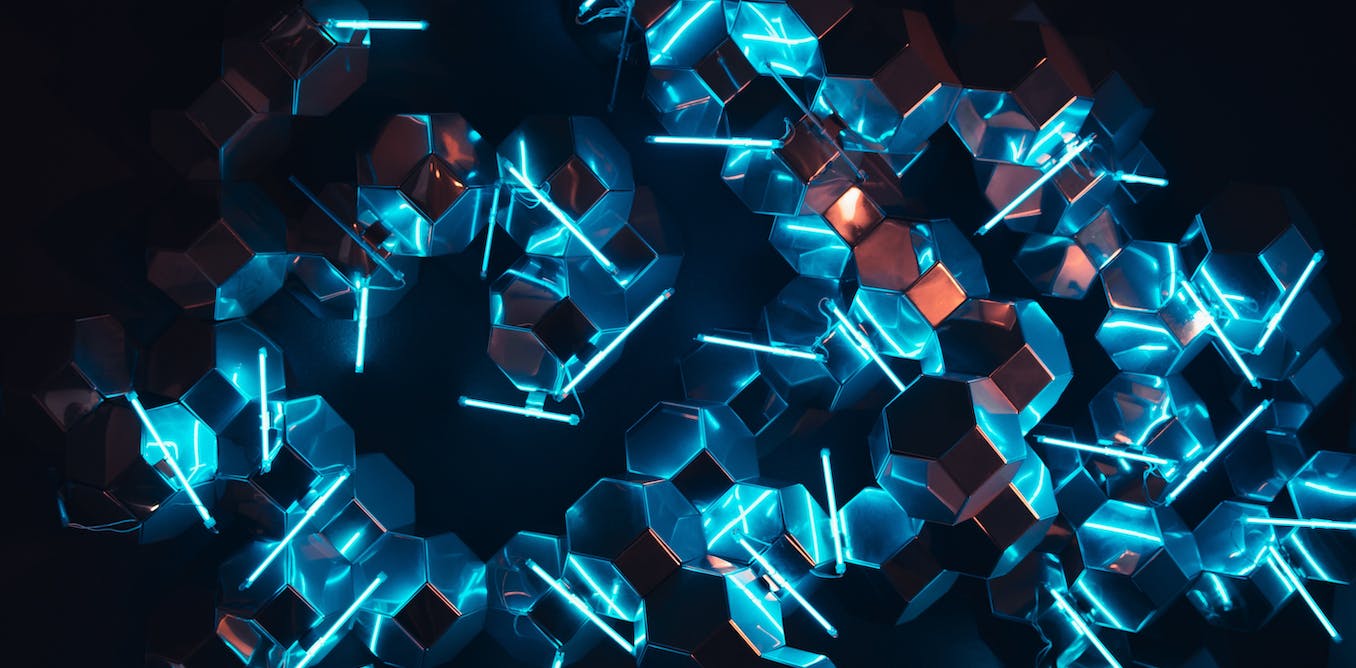
The energy consumption of large computers is very high – so what about future quantum computers? maximalfocus/Unsplash, CC BY
Quantum computers have attracted considerable interest of late for their potential to crack problems in a few hours where they might take the age of the universe (i.e., tens of billions of years) on the best supercomputers. Their real-life applications are manifold and range from drugs and materials design to solving complex optimisation problems. They are therefore primarily intended for scientific and industrial research.
Traditionally, “quantum supremacy” is sought from the point of view of raw computing power: we want to calculate (much) faster.
However, the question of its energy consumption could also now warrant research, with current supercomputers sometimes consuming as much electricity as a small town (which could in fact limit the increase in their computing power). Information technologies, at their end, accounted for 11% of global electricity consumption in 2020.
Why focus on the energy consumption of quantum computers?
Since a quantum computer can solve problems in a few hours where a supercomputer might take several tens of billions of years, it is natural to expect it will consume much less energy. However, manufacturing such powerful quantum computers will require that we solve many scientific and technological challenges, potentially over one to several decades of research.
A more modest goal would be to create less powerful quantum computers, capable of solving computations in a time relatively comparable to supercomputers, but using much less energy.
Google, Amazon, Microsoft and IBM are some of the tech giants to have taken an interest in quantum computing.
Graham Carlow
This potential energy benefit of quantum computing has already been discussed. Google’s Sycamore quantum processor consumes 26 kilowatts of electrical power, far less than a supercomputer, and runs a test quantum algorithm in seconds. Following the experiment, scientists put forward classical algorithms to simulate the quantum algorithm. The first proposals for classical algorithms required much more energy – which seemed to demonstrate the energy advantage of quantum computing, but they were soon followed by other proposals, which were much more energy efficient.
The question of the energy advantage is therefore still open to question and is an open research topic, especially since the quantum algorithm performed by Sycamore has no identified “useful” application to date.
Superposition: the fragile phenomenon at the heart of quantum computing
To know whether quantum computers can be expected to provide an energy advantage, it is necessary to understand the fundamental laws according to which they operate.
Quantum computers manipulate physical systems called qubits (for quantum bits) to perform a calculation. A qubit can take two values: 0 (the “ground state”, of minimum energy) and 1 (the “excited state”, of maximum energy). It can also occupy a “superposition” of 0 and 1. How we interpret superpositions is still the subject of heated philosophical debates, but, to put it simply, it means that the qubit can be “both” in state 0 and state 1 with certain associated “probability amplitudes”.
Thanks to these probabilities, we can greatly simplify the principle of the quantum computer by saying that it implements algorithms that perform calculations on several numbers “at once” (in this case 0 and 1 at the same time). This advantage becomes clear when the number of qubits is increased: 300 qubits in superpositions are capable of representing 2 to the power of 300 states at the same time. As an example, this is approximately the number of atoms in the observable universe – so representing so many states at once on a supercomputer is completely unrealistic.
Erik Lucero, lead engineer of Google Quantum AI, stands beside a quantum computer in Goleta, California in September.
Frederic Brown/AFP
However, the foundations of quantum theory tell us that if the values of these probability amplitudes are “measured” by another physical system, then the superposition is destroyed: the qubit relaxes to the value of 1 or 0, thus introducing an error into the calculation.
One concrete example of such a destruction is when the qubit absorbs a photon (a particle of light that is a small packet of energy). If this is the case, it is because it was not in its maximum energy state (since it can absorb energy, that of the photon). The photon, and therefore through it the “environment” of the qubit has therefore indirectly “found” the value of the amplitudes, which destroys the superposition. This is called “decoherence”.
[Nearly 80,000 readers look to The Conversation France’s newsletter for expert insights into the world’s most pressing issues. Sign up now]
Generally speaking, the challenge is to ensure that the qubits are sufficiently isolated to avoid any information leakage: we can’t allow a photon or another particle to disturb our qubit. This is a challenge because the qubits must also be controllable: they cannot be completely isolated.
This lack of protection is the main source of error in qubit-based calculations. For example, one of the most mature qubit technologies runs into an error every 1,000 operations. When you consider that it takes 10¹³ operations for a typical quantum algorithm, you can see that this is far too many.
Preserving superpositions has an energy cost
The energy cost of computing a quantum computer will mostly come from this need for “protection of the quantum data”. For example, it is often necessary to set the qubit environment close to absolute zero (-273°C) to ensure that no photons populate this environment, avoiding the problem mentioned above. This is a very energy-intensive process.
Some other techniques, such as quantum error correction, also preserve quantum information, and can improve the fidelity of operations. However, in addition to the challenges they raise, these techniques also incur a very high energy cost because they involve error detection algorithms, or additional qubits for error detection, etc.
In short, the more accurate we want an operation performed on a qubit to be, the more it will have to be protected, and the more energy we will have to spend for that. There is a very strong link between “error rate” and “energy” in quantum computing. Understanding this link precisely may then allow the design of a very energy efficient computer.
Is an energy quantum advantage possible?
Some theoretical studies have been able to calculate the energy cost necessary for the realisation of quantum computers, but in a non-optimised regime, notably not exploiting the link between error rate and energy, and often with a simplified model of the computer.
Exploiting this link can lead to powerful optimisations reducing the energy cost of algorithms. In practice, this requires an interdisciplinary approach including the understanding of the fundamental phenomena inducing decoherence, the modelling of quantum error correction algorithms and codes as well as the whole “engineering” part necessary to control the qubits. One can then calculate the minimal energy cost needed to solve different problems, while aiming at an error probability for the algorithm considered as “acceptable”.
A wafer of a D-Wave quantum computing system.
Creative Commons Attribution
As we have seen, for qubits of excellent quality (i.e., of a quality that is still out of reach in practice today), there are tasks for which the quantum computer could spend one hundred times less energy than the best current supercomputers for a comparable calculation time (comparable in the sense that both would be able to solve the task in a reasonable time). This energy gain of a factor of 100 is also indicative: one could imagine saving more energy by carrying out additional optimisations.
This is because a quantum computer computes using fundamentally different processes to a quantum computer: the former manipulates qubits and the latter bits. Thus, for the same task and even for the same computing time, the number of operations can be drastically different. Moreover, an operation performed in a quantum computer will involve physical processes that are radically different from implemented on a supercomputer. These two remarks taken together imply that, conceptually, even at equal computation time, even if a quantum logic operation consumes more energy than a classical logic operation, the smaller number of quantum logic operations may mean that the quantum computer will ultimately be much more energy-efficient.
Of course, this example comes from theoretical calculations, based on sometimes highly optimistic assumptions. However, it does seem to indicate that one of the primary advantages of quantum computing may well be energetic before it is computational.
Marco Fellous-Asiani does not work for, consult, own shares in or receive funding from any company or organisation that would benefit from this article, and has disclosed no relevant affiliations beyond their academic appointment.